On Tuesday, MIT physics professor Nikta Fakhri gave a guest lecture on nonequilibrium processes in living matter and how they assist in making life possible.
What is life? There is currently no answer to this question, and perhaps we will never obtain one. Nonetheless, various scientific fields have different ideas about how humans could go about investigating this query. One of the areas with some of the most interesting theories and experiments is biophysics. In their eyes, life is a macroscopic process. To be more specific, its characteristic feature is its ability to avoid thermodynamic equilibrium: that is to say, death. How exactly this nonequilibrium is achieved is an issue that plagues biophysicists across the world. Nikta Fakhri, a professor of physics at MIT and a renowned expert in the field, attempts to tackle this problem by probing biological systems to identify the aspects of their non-equilibrium. On Tuesday, she gave a guest lecture at the Columbia Chemical Engineering colloquium, speaking about her extensive work on this subject.
To analyze what exactly the key degrees of freedom of living matter are, scientists analyze a plethora of different scenarios. In the lecture, Dr. Fakhri presented three cases their group had studied, ranging from the intracellular to organismal levels. The most microscopic of these situations analyzes the breaking of time reversal symmetry. Time reversal symmetry refers to the tendency of most physical laws to not have a preferred direction in time—that the future is essentially the same as the past. Time is an “illusion” for these processes, referring to the fact that the only thing distinguishing the forward flow of time is the increase of entropy. Entropy is also what can be used to identify nonequilibrium processes that break time reversal symmetry. Actin and myosin are two examples of proteins involved in processes between cells, and both engage in various “fluctuations” during processes. The Fakhri group developed tiny infrared fluorescent granules that allowed them to generate maps of these fluctuations and their noise. This noise consists of several parts, including thermal and active components, but their quantities are established. The group was looking for something different, and they managed to find it. On long time scales, thermal and active noise eventually becomes less relevant, and noise from nonequilibrium activity can be identified. Using various algorithms, they were able to find that at a certain time, the irreversibility of the system (its distance from equilibrium) reaches a peak. This allowed them to go back to the original question and conclude that the system was using nonequilibrium processes to regulate the mechanics of cells and the processes between them.
This is only one case in which it is clear that a nonequilibrium process is contributing to the activity of living matter, and the group has several other projects running in tandem. The second of these that was discussed involved processes that occur on a cellular scale: protein patterns. These patterns represent surface contractions and were characterized by the group on starfish egg cells. These contraction waves were found to be induced by the signaling protein Rho-GTP and were seen to exist no matter what shape of egg cell was analyzed. But even more importantly, the Fakhri group found that the dynamics of Rho-GTP were in turn caused by another protein: Ect2. Protein patterns were being caused by other protein patterns. Using this exciting discovery, the group managed to manipulate the dynamics of Rho-GTP (which is related to Ect2 through a temporal derivative) by varying the expression of Ect2. In these experiments, they found that the complexity of the generated patterns increased with heightened cellular activity. They then constructed a mathematical model known as a phase field and saw that topological defects, which are fingerprints of broken symmetry, correlated with the cellular activity and consequently the pattern complexity. They even managed to control the generation of these defects by using light and succeeded in changing the kinetics and shapes of the patterns. This was once again a confirmation that nonequilibrium processes were participating in living processes.
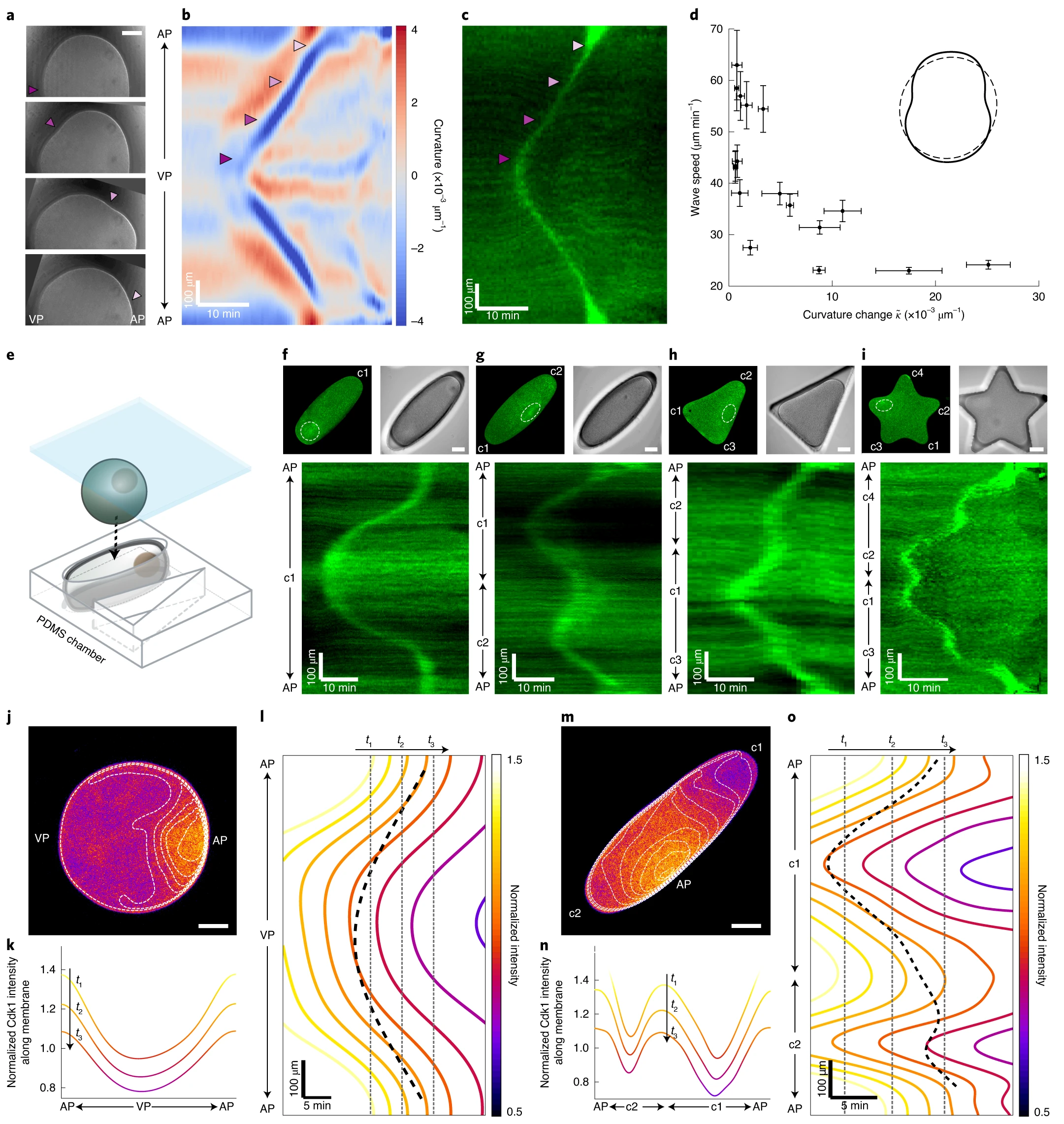
The last of the projects discussed during the colloquium existed on the largest scale and involved starfish embryos as a testing site. Certain groups of active matter have been found to be able to self-organize into living crystals, which breaks two types of symmetry: time reversal and chirality. Chirality is an important idea in various branches of science that has to do with an object’s asymmetry. The group observed this phenomenon themselves with the starfish embryos, which used fluid flows generated by their cilia (hair-like extrusions) to “swim” and assemble into living chiral crystals. This process happened over several days, and on a scale large enough to be observable by human eyes. They closely analyzed this process and the microscopic interactions within it, including the fluid mechanics and elasticity, and also concluded that irreversible processes were participating in the control of the active processes.
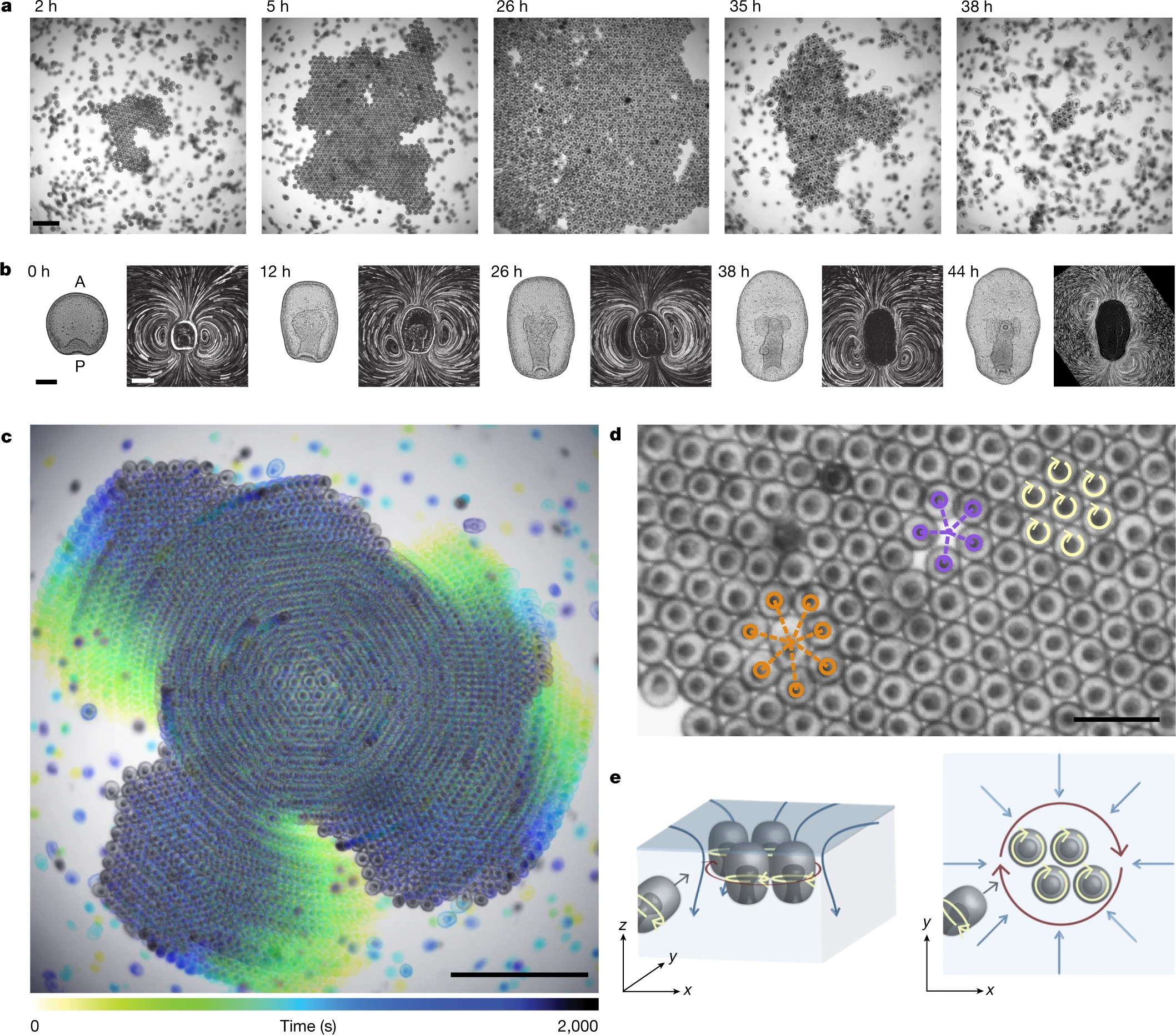
Though they already have extensively studied many cases, Nikta Fakhri and her group are not finished. In the future, they hope to pursue projects such as testing the response of chiral crystals within a contained area, actuating the crystals by implanting ferrofluids, and analyzing the role that geometry and phase transitions play in active processes. In the end, what can we conclude from this about life? It is clear that the principles of non-equilibrium systems can provide clues to the bigger questions, and allow scientists to learn more about living matter. However, what Dr. Fakhri says could be even more important, is that the study of these systems could lead to the rise of new types and areas of physics in the future.
Header via MIT News
Protein pattern image via M.C. Wigbers et al 2021 Nature Physics
Living chiral crystal image via T.H. Tan et al 2022 Nature