On Wednesday, Charlie Bonkowsky attended “Dwarf Galaxy Archaeology with the Rapid Neutron-capture Process,” a colloquium presented by Dr. Alex Ji of the University of Chicago.
Any element heavier than hydrogen and helium had to be forged in the cores of stars. The carbon in your body, for example, was probably created in the dying throes of a star about the same size as our sun. Heavier elements, like iron or aluminum, are created when more massive stars die and explode in violent supernovae.
But there’s long been uncertainty about the creation of the very heaviest elements—especially with the ones towards the bottom of the periodic table.
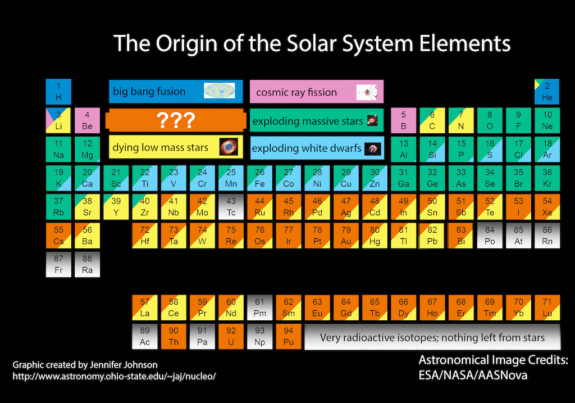
We can create these lower-grouped elements in the lab through a process known as rapid neutron capture, or the r-process. Neutrons are rapidly added to the nuclei of lighter elements, which then undergo beta-decay to the stable isotopes of heavier elements. But this is a complicated series of steps, and so the question remains: what astronomical phenomena might create these r-process elements, and how can we observe them?
On Wednesday, Dr. Alex Ji spoke about his work with dwarf galaxies and how he used them as case studies, so to speak, for examining this sort of stellar nucleosynthesis. But the first step, as he said, was finding these dwarf galaxies.
The most famous nearby dwarf galaxies are the Large and Small Magellanic Clouds, discovered in 1916. But our own galaxy, the Milky Way, contains remnants of dozens of dwarf galaxies—some still intact, others fully disrupted, others strung out by gravitational forces into long streams of stars. Most, Ji said, aren’t visible just by looking at the sky with conventional telescopes—including his favorite dwarf galaxy, Reticulum II.
(Side note: this is one of the reasons I love going to astronomy colloquia: where else are you going to find people with a favorite dwarf galaxy? And one that you can’t even see at that?)
Instead, using data from the Sloan Sky Survey, astronomers can measure the distance to every star, how fast it’s moving around the Milky Way, and more. They can then predict that groups of stars which are all similar distances away from us, moving at about the same speed, probably belong to a dwarf galaxy which has been swallowed by the Milky Way. Based on the age of these stars, astronomers can also estimate when these galaxies stopped forming new stars—which will prove to be a very useful piece of knowledge to have.
So how can we use dwarf galaxies to study the creation of r-process elements? Ji told us that there are two main theories: that r-process elements are rarely formed in the core-collapse supernovae of massive stars (CCSNs), or through the collision of two neutron stars (NSMs). And in 2017, astronomers got a windfall: a neutron star merger picked up by LIGO and by conventional telescopes, in which the characteristic red light curves of r-process elements being created was seen. So we know that NSMs produce r-process elements—but are they the only thing that does so? And how do we find that out without waiting to get extremely lucky?
Here’s the key part of Ji’s work: CCSNs and NSMs produce r-process elements on different timescales. CCSNs should produce r-process elements on a timescale of tens of millions of years after star formation, while NSMs should do so on a timescale of hundreds of millions to billions of years.
First, Ji looked at small, ultra-faint dwarf galaxies like Reticulum II, which contain less than 100,000 sun’s worth of mass and are usually gravitationally dominated by dark matter. The benefit of studying them, he said, is actually the rarity of r-process events. Say you have 10 galaxies, each with a mass of 10,000 suns. Based on what we can assume, we should only see one of them with any r-process event having occurred there—and that’s what we do see! Reticulum II is his favorite dwarf galaxy because its stars contain hundreds of times more elements like europium and barium than any other ultra-faint dwarf galaxy, confirming that there must have been a single rare r-process event that took place in this dwarf galaxy and none of the others.
By measuring the age of the stars and how many of them contained these rare metals—a process which Ji said “turned out to be harder than I thought,” he found that it could have taken no longer than 500 million years after star formation for this event to occur. The number is better than what astronomers had previously but isn’t the smoking gun that would prove CCSNs can also serve as r-process events.
It does tell us something interesting about dwarf galaxies, though. All of these r-process metals in Reticulum II are well-mixed: there’s the same distribution in every star. This implies that star formation in dwarf galaxies is “bursty”—there’s a “burst” of star formation, and then a time during which no more stars are formed. Simulations show that it would take more than 100 million years for these metals to disperse evenly throughout Reticulum II, so the “bursts” of star formation must be separated by at least 100 million years. This, he said, is the first observational evidence of this kind of cyclic star formation in such ultra-faint dwarf galaxies.
Second, Ji looked at large dwarf galaxies which have been completely subsumed into the larger Milky Way, as a simulation like the one below shows.
These include the dwarf galaxies Gaia-Sausage-Enceladus (GSE) (great name) and Kraken, which are of similar mass but stopped forming their stars at different times. Kraken formed stars for only 2 billion years before it stopped, while GSE formed stars for about 3.6 billion years.
Stars in GSE contain more r-process elements than Kraken. This serves as another confirmation that NSMs must be an r-process source, because they’re the only process which would continue to enrich stars after billions of years. But Ji added the caveat that there must still be an (as yet unconfirmed) quick r-process source, because there are no stars without any europium whatsoever. NSMs are the best-known source right now, but they cannot be the sole source.
What does the future hold? For Ji, the answer’s easy: a lot more dwarf galaxies. Through new telescopes and new collaborations, like the Southern Stellar Stream Spectroscopic Survey Collaboration at the Anglo-Australian telescope, he’s hopeful that we’ll find the faintest disrupted galaxies: low-mass galaxies completely shredded by the Milky Way. “You might think that’s impossible,” he said, “but I think they’re possible to find.”
And in the coming decade, he is hopeful we’d find all dwarf galaxies, whether intact or disrupted, within and around the Milky Way. And with that many dwarf galaxies, we’d have a cosmologically representative sample—a window into the broader universe from the galaxies around us.
Dwarf galaxies via ESA/Hubble and NASA
Periodic table via Jennifer Johnson