On Wednesday, Staff Writer Charlie Bonkowsky attended the colloquium “Moving Closer to a Detection of nHz-frequency Gravitational Waves with NANOGrav,” by Scott Ransom, a founder of the NANOGrav project.
Supermassive black hole mergers are some of the most destructive events in the universe: two objects, each with the mass of billions of stars, slowly spiraling in towards one another until the inevitable, catastrophic collision. But we here on Earth can’t see them. To detect such huge, faraway events, we need a “galactic-scale detector”—and that’s NANOGrav.
We can’t search for supermassive black hole collisions using standard telescopes—they’re simply too faint, and, for most of their lifetime, won’t really be emitting any light. But they will be emitting gravitational waves, ripples in spacetime which travel outwards at the speed of light.
The first detection of gravitational waves only occurred in 2016, at the Laser Interferometer Gravitational Observatory (LIGO)—it’s a very new branch of science. At LIGO, a beam of light is split by a partially reflective mirror: half the light travels down each of two long arms, where it’s reflected back to a single detector. Normally, the beams are tuned to destructively interfere with each other, and the detector will see no light. But when a gravitational wave passes by, it stretches spacetime, changing the length of the two arms so that the light beams fall out of phase with one another and create a ‘flicker’ at the detector.
NANOGrav works similarly, but on a much larger scale. We can’t set up lasers to span the galaxy, but we have the next best thing: pulsars. Pulsars are a special type of neutron star which a) spin very quickly, and b) emit jets of electromagnetic ration from their poles. NANOGrav is focused on millisecond pulsars (MSPs), which spin quickly enough to complete an entire rotation in—well, just milliseconds (the fastest known MSP spins 717 times per second!). And, more importantly, they always spin at the same rate, changing by less than a second over ten billion years, which can be analyzed and then predicted to frightening accuracy.
The timing of many MSPs is collected by NANOGrav researchers. Then, like LIGO, any disturbance in spacetime between Earth and the pulsar causes the light to arrive slightly earlier or later, and scientists can analyze those timing shifts to pick out the gravitational wave signature.
As Ransom said, the NANOGrav project has been running for about 13 years now, using a variety of telescopes. The Arecibo telescope in Puerto Rico, which collapsed in 2020, was one of the linchpins of the project—which made it a tragedy to lose. Fortunately, they were able to ramp up the use of the Green Bank Telescope in West Virginia so as not to lose too many observations, and make use of a new Canadian radio telescope—CHIME—for their most low-frequency observations.
And what have they found in 13 years of data?
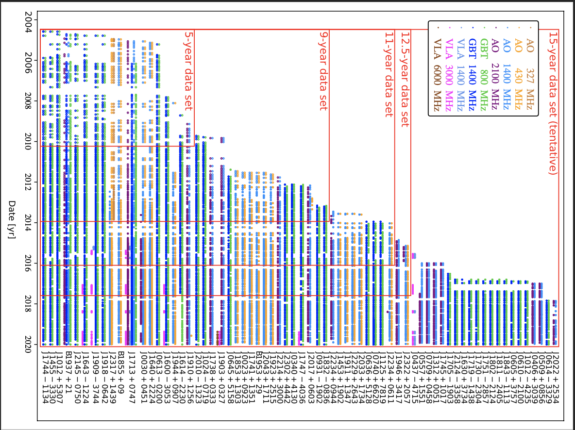
First: they haven’t found nano-Hz gravitational waves (those signatures of supermassive black hole mergers). Yet. To show that they’ve detected gravitational waves, the difference between expected and actual arrival time of pulsar signals should follow the characteristic Hellings-Down curve (the blue line on the plot below). Right now—their timing residuals don’t do that. But Ransom was hopeful: the data gets better every year and with each new pulsar added, he said, and already the differences between the 11-year (above) and 12.5-year (below) data set are clear. Based on modeling, the first gravitational waves should be detected by NANOGrav before the end of the decade.
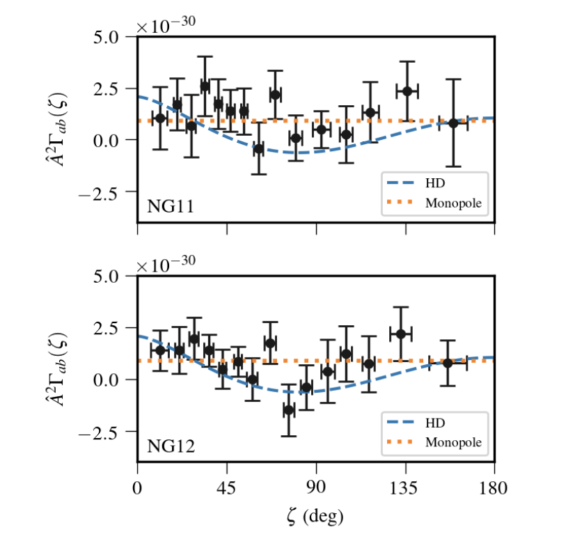
Second: they’ve found a lot of other cool stuff! Pulsar timing arrays receive and require incredibly precise data, much more than even other space projects. When looking at their 11-year data, he said, they found that JPL’s catalog of every planet in the Solar System’s location and orbit wasn’t exact enough—the same JPL that, during that time, was launching and landing Mars rovers on a dime. They’ve also found the most massive neutron star known, and put constraints on the neutron star equations of state, which physicists are, apparently really excited about.
What could be more exciting than that? “Even better than the theory papers,” Ransom said, “was that we made it into xkcd.”
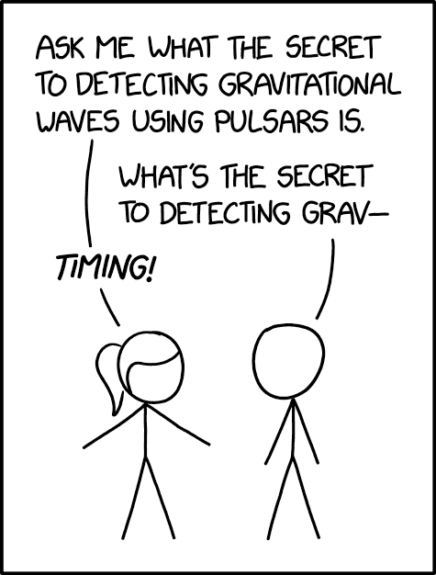
Pulsar Timing Array Cartoon via NANOGrav
NANOGrav Epoch Plot via NANOGrav
Hellings-Down Plot via NANOGrav Collaboration
Gravitational Wave Timing via xkcd