On Wednesday, Staff Writer Charlie Bonkowsky attended two talks at the Astronomy Department: on binary star systems and their evolution, by Katelyn Breivik, and on high-energy black hole flares, by Bart Ripperda.
Every one or two weeks, Columbia’s astronomy department hosts talks and colloquia from researchers across the country. I’ve covered a fair number of them for Bwog—all of them on super cool topics like gravitational waves or exoplanet surfaces—and sometimes I wonder why I’m one of the few undergrads there. Why don’t more of us take advantage of these opportunities? Then sometimes I see that no, these are definitely talks aimed at people who Know Physics.
This was one of those times.
The first talk, by Katelyn Breivik, focused on understanding the evolution of binary-star systems. Breivik described an evolutionary process beginning with two detached stars, which, as one star strips away the mass of another, transitions into a single luminous star orbiting a black hole. From there, the black hole begins to strip mass from its companion, resulting in two black holes orbiting one another. Eventually, as gravitational-wave detectors like LIGO observe, these two black holes spiral into one another and collide.
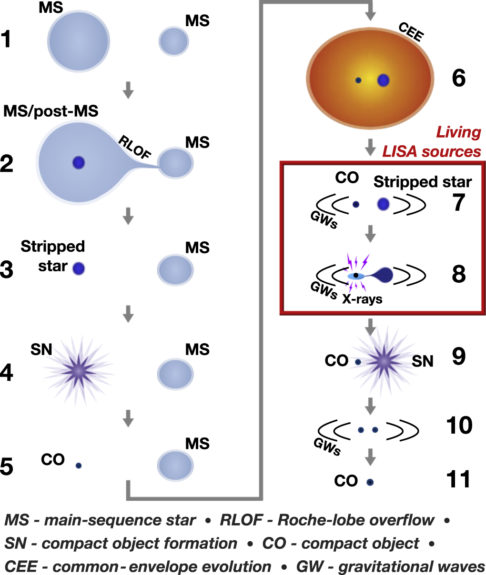
Breivik is a theorist, and so she works mostly in simulations—specifically forecasting the number of black hole–star binaries we should be above to observe in our own galaxy. The primary telescope for those observations is Gaia, an ESA space observatory. Her simulations take on a lot of different parameters: some stellar, like stellar formation in the Milky Way based on observed metallicity; others logistical, like the size of orbits or star brightness that Gaia can actually observe.
But from there, it’s a little tricky. In a data-rich environment, she said, ideally the theory of binary-system evolution would lead to new models, which could then be checked against observed populations and the theory updated. But there are few binary systems with a star orbiting a black hole; in fact, as of the date of her talk, there are only two. VFTS 243, in the Large Magellanic Cloud, and Gaia BH 1. And even those are problematic for the modes: VFTS 243 is outside the Milky Way, so it’s not part of her galaxy-wide model; and Gaia BH 1 is just plain weird.
The system is composed of a ten-solar-mass black hole and a one-solar-mass star, orbiting around one another every 185 days. And “that’s a little zany,” she said, because of what it implies about the common-envelope process from which one star took mass from the other. That process must have been extremely efficient, more than anything else their model predicated, and featured an extreme mass difference between those stars at the beginning: something like 70-to-1. Her paper, Breivik said, concluded simply that this challenges existing binary-evolution models, but it’s hard to know without more data. Two binary systems with a black hole and a star aren’t really enough to define nor disprove a model.
Next up was Bart Ripperda, whose talk focused on understanding the origins of high-energy black hole flares. The first detections of black holes, he said, were actually the detection of enormous jets of radio waves, which scientists realized later were being powered by supermassive black holes at their source. There’s been a lot of research into those jets and the physics behind them—he wanted to focus, then, on something new.
Black holes and their associated jets often show high-energy, non-thermal flares from their central core or along their jets, and their origins are still unclear. One common analogy, he said, was solar flares, which is the conversion of magnetic energy into kinetic energy…an analogy which, it so happens, is very useful.
First, however, we needed to discuss plasma physics at the black hole’s event horizon. However, the magnetohydrodynamic description of plasma, and the way relativistic plasma works differently from regular plasma, is somewhat above my pay grade. The fact that relativistic plasma is highly magnetized and collisionless makes it easy to accelerate, and thus, a likely power source for the high-energy flares—but magnetized plasma, in itself, does not radiate. So there must be another step to the process.
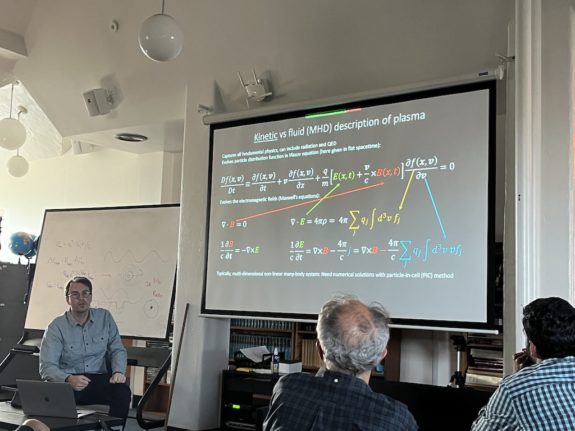
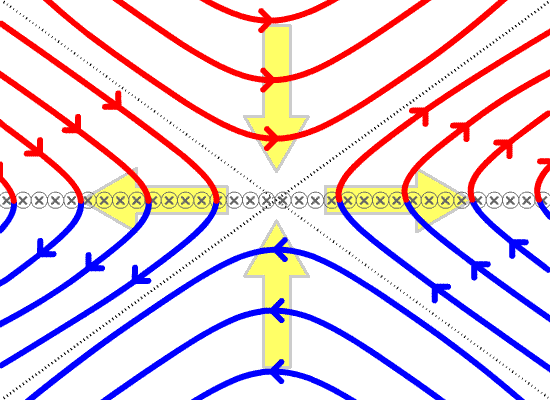
Let’s return to solar flares. In new research, astronomers have been able to look extremely closely at each step of a solar flare, which involves a process known as magnetic field reconnection. Two magnetic field lines are pressed together and then burst outwards—but what closer observation showed is, on the Sun, the formation of tiny bubbles of non-magnetized plasma where the field lines met. And in relativistic plasma, simulations have shown, those tiny bubtbles will combine with each other and grow, providing a perfect source for massive, high-energy flares. Therefore, Ripperda proposed, magnetic reconnection near a black hole’s horizon could be the key to understanding these flares.
Working from that model, he and his team were able to produce detailed models of reconnection close to the horizon. As a black hole sucks in gas and dust and other matter, it also ‘sucks in’ its own magnetic field lines, which are scrambled and pushed closer together in the process. And as in the simplified animation above, this transforms its horizontal field into a vertical field briefly, causing everything outside a certain radius to be ejected outwards: a flare.
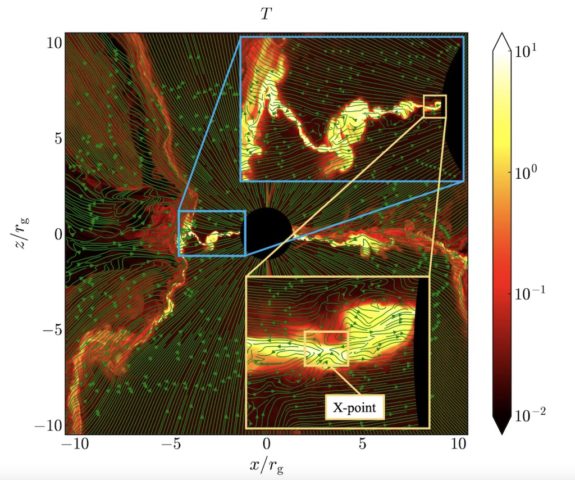
Lastly, he said, they discovered that this process of flaring isn’t just something that black holes do: it’s actually essential to the way black holes work. If not for the flares intermittently pushing everything away from the black hole, the black hole would actually stop growing: its accretion disk, the mass of gas and dust pulled in by its gravity, would choke it off.
And the cool part? Despite much of this happening at the small-scale, in the formation of plasma bubbles and movement of magnetic field lines…we can still see it happening. Radio telescopes have seen indications of the magnetic field around the supermassive black hole M87, and, with the new James Webb Space Telescope starting its observations, there’ll soon be lots more to see.
Black hole field lines and simulation via B. Ripperda et al 2022, ApJL
Binary star evolution via K. Breivik et al 2020, ApJL
Magnetic field reconnection via Wikimedia
Ripperda presentation photo via Charlie Bonkowsky